Agent Any force or process that accumulates and/or modifies biological remains; agents may be biological entities or physical forces such as chemical reactions or gravity. assemblage A collection of objects that occurs within the limits of a definable space or locality.
Crystallinity The size and orderliness of the crystalline lattice of a material.
Density In vertebrate taphonomy, two distinct uses of the concept to describe skeletal materials are important: structural density refers to the variations in the porosity of a material in relation to volume and holding material class constant (e. g., bone as opposed to tooth enamel); mineral density instead refers to the relative proportion of organic and inorganic components in a material (e. g., mature mammalian tooth enamel is about 95% calcium phosphate mineral, whereas mature bone is composed of about 65% of the same mineral).
Diagenesis Chemical transformations of materials postmortem, including mineral dissolution, recrystallization, and replacement. dissolution Chemical breakdown assisted by water, most often provoked by an imbalance in the pH of a sedimentary environment.
Equifinality Similar consequences or signatures arising from two or more distinct agents, processes, or causal pathways. fossilization Any process by which the original components of a biological structure are replaced or altered by dissolution, mineral precipitation, or both, but preserving the appearance or surfaces of the original structure. inorganic In biological remains, the component of a material composed of mineral compounds. in situ attrition The loss by deletion or decomposition of materials from a locality where they accumulated previously. organic In biological remains, the component of a material composed of organic compounds such as protein, lipids, and sugars.
Phytoliths Silicious structures formed within the soft skeleton of plants, some of which are diagnostic of broad plant taxonomic groups.
Porosity The proportion of the volume of a material taken up by holes, tubes, or irregular voids; in zooarchaeology, the structural density of material traditionally is measured as the relation between volume and weight and more recently by application of photon densitometry and computed tomography techniques. specimen Any discrete object, whole or broken. superficial damage Visible modifications of the surface of a specimen such as etching caused by contact with plant roots,
Burning by fire, exfoliation provoked by atmospheric weathering, gnawing damage by animals, cut marks, or battering from tools.
Uniformitarianism A highly influential analytical concept of time-independent process originating in geology (C. Lyell) but integral to all historical or interpretive sciences, including taphonomy; assumptions of constancy, regularity, or consistency of key processes or structures in nature, biological and physical.
Zooarchaeology The study of animal remains from
Archaeological sites; analyses are most often organized around economic questions but may also include uses of animals or parts of animals in ritual contexts or as sources of raw materials in technology.
‘Taphonomy’ represents a family of scientific methods developed principally in the service of palaeontology, archaeology, and forensics. It is concerned foremost with historical reconstruction of past events or processes that lead to the formation of a collection of associated objects, most often biological remains. Taphonomy first emerged as a discipline in the nineteenth century, mainly among European palaeontologists inspired by uniformitarian approaches from geology, with some contributions by American researchers. The discipline expanded rapidly in the second half of the twentieth century in response to new questions about depositional environments, consequences of differential decay, and the forces of accumulation and modification of animal and plant remains in sedimentary records.
J. Weigelt is recognized as a formative influence in vertebrate taphonomy, not least for his notion of ‘bio-stratonomy’, the study of the processes that operate on organismic remains from the time of death to their integration into the geological record. The broad applicability of Weigelt’s work stems from his preoccupation with decay and burial processes, which he observed in contemporary environments as a means for understanding the formation of fossil records. R. Richter also employed uniformitarian concepts in his version of actualistic paleontology (aktuo-palantologie), which focused on the accumulation and alteration of skeletal materials prior to fossilization. A. H. Muller instead examined the effects of diagenesis (fossildiagenese) following the burial of organismic remains - geochemical transformations including mineral replacement that occurs during fossilization.
Early developments in the field were highly varied and problem specific. I. A. Efremov is credited with synthesizing the diverse aspects of experimental, actualistic, behavioral, mechanical, and geochemical research into the discipline now called taphonomy. Prior to the 1970s, two scientific traditions existed within the discipline, one in palaeontology and the other in archaeology (mainly zooarchaeology). The goals of analysis and the methods commonly employed in the two traditions overlapped greatly, however, and considerable cross-fertilization is evidenced in the literature particularly after 1960. Convergence of the taphonomic traditions in palaeontology and archaeology is readily apparent in the works of C. K. Brain, B. Kurten, G. Haynes, and others, who applied taphonomic methods to problems of Pleistocene animal behavior and human evolution.
Biological remains that frequently come under ta-phonomic investigation are vertebrate skeletons, mol-lusk shells, and plant pollen and phytoliths (see Pollen Analysis; Phytolith Analysis). An essential distinction is drawn analytically between organic and inorganic components of biological remains, and taphonomic methods and techniques tend to specialize within these material categories. The chemical make-up of a material greatly influences its susceptibility to decomposition, and the organic and inorganic components are nearly opposite in their susceptibility to variations in sediment pH (acidic to basic chemical conditions). The geological conditions that affect the preservation of organic and inorganic components of materials in sediments therefore are also important for estimating the extent of preservation bias.
This essay deals mainly with the taphonomy of vertebrate and mollusk skeletal remains, particularly the inorganic components. In vertebrates, the inorganic fraction of skeletons is dominated by calcium phosphate minerals and those of mollusk shells by the carbonate minerals, aragonite and calcite. It is the mineral component of skeletons that tends to retain diagnostic macrostructures postmortem.
The taphonomic methods used by zooarchaeologists, palaeontologists, and forensic scientists benefit from reference cases, constants, and natural principles drawn from both the physical and biological sciences. Inferences about past events are strengthened significantly by reference to independent recent cases of known history, whether derived from natural or experimental contexts. These sources of reference provide the inferential bridges between the observations about a collection of older materials and the processes responsible for their formation. More than one process may produce the same kind of pattern or signature, however, and thus referents or analogs are most valuable in taphonomic studies for illuminating the range of potential causes. Reference data may also be useful for eliminating some potential explanations, but their value for this purpose is more limited.
Common referents in taphonomic research include the typical age composition of organism populations, the duration of each reproductive generation (turnover rate), organism anatomy and physiology. Also important are experimental data on size and shape sorting of organismic remains by water currents or gravity, chemical reactions provoked by changes in the pH of sediments or water surrounding the remains, and superficial damage resulting from chewing or digestion by other animals, plant roots or atmospheric exposure (‘weathering’). It is assumed that the reference data employed in a study accurately represent a timeless (uniformitarian) process or a constant property of a physical structure. Examples of time-independent or ‘uniform’ processes include the effects of gravity and biological decay. Examples of structural constants include simple yet crucial expectations of the number of limbs in the complete skeleton of a species, and, more generally, the chain of predator-prey relationships (trophic hierarchy) in a food web.
Taphonomists tend to focus their studies on assemblages of specimens rather than on the history of isolated finds, as the former is a richer source of information about the past. Most primary observations nonetheless are taken specimen by specimen, in addition to observations about sedimentary context. The data are then examined in aggregate for diagnostic patterns of damage, spatial distributions, and other indications. Because the history of each object in an assemblage can differ from the next, overall assessments of assemblage formation tend to be complex and are built from arguments of probability. Taphonomists therefore seek to identify not only the identity of dominant and secondary agents of accumulation, modification, and preservation for the assemblage as a whole, but also the sequence of agent effects (exemplified in Figure 1). The span of time over which an assemblage forms may vary from a few seconds to thousands of years. The longer the formation time of an assemblage, the greater the likelihood that multiple factors have contributed to shaping the overall character of that assemblage.
The cause(s) of assemblage accumulation and/or modification normally is assigned to one or more agents. These agents can be biological, such as animals that prey on other animals or collect their bones, or physical forces such as gravity or solar radiation. Analyses of superficial damage on skeletal specimens most
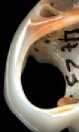
Figure 1 Examples of singular and sequential damage on marine shells: (a) wave-induced abrasion of fractured shell; (b) bore hole of molluskan predator in prey; (c) damage sequence that began with death caused by molluskan predator followed by wave-induced abrasion.
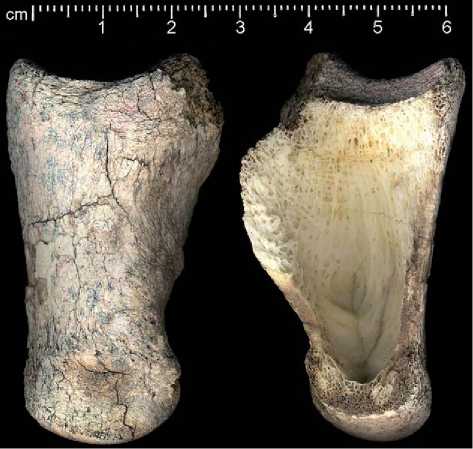
Figure 2 Atmospheric weathering of bones leaves characteristic traces, affecting the exterior surface first; weathering eventually may reduce an entire specimen to dust if exposed on the ground surface for long periods; burial in sediments tends to prevent or arrest the weathering process.
Often focus on the positive indications of alteration. Weathering damage from atmospheric, especially solar, exposure (Figure 2) is indicative of the rates of burial or vegetal overgrowth, because the process generally ceases once the specimen is sheltered from the elements. Gnawing damage from carnivorous animals (Figure 3 ) or rodents (Figures 4 and 5) may imply that specimens lay exposed and available to consumers for extended periods. Burning damage on bones in archaeological sites usually indicates human activities, and variation in the distribution and intensity of burning damage in these contexts can be used to diagnose fire-centered activity areas, intentional waste disposal, or cycles of hearth rebuilding, since the act of cooking seldom results in extensive or repeated burning of bones directly. Tool marks on bones resulting from contact with the sharp edges of chipped stone tools (Figure 6) or blunt hammers (Figure 7) are also diagnostic of human activities and, in many cases, provide additional layers of information about butchering, marrow extraction, or fat-rendering practices. Abraded or polished surfaces on bones or resistant plant parts may develop under a wide variety of conditions. The distribution and microscopic characteristics of abrasion and polishing damage often allow researchers to determine their cause. Rolling of materials down a dry slope, trampling by large animals, rounding or etching from digestion in the gut of a consumer all tend to affect the entire surface of a specimen while producing distinctive textures. Other agents produce asymmetrical or localized abrasion or polishing. Bones half buried in a stream channel, for example, will be abraded locally as the water pushes sediment particles across the surfaces that remain exposed. Microscopic polish (use-wear traces) found on the working surfaces of bone, antler, or wooden tools (Figure 8) are nearly always localized and position specific. Tool function sometimes can be determined from a combination
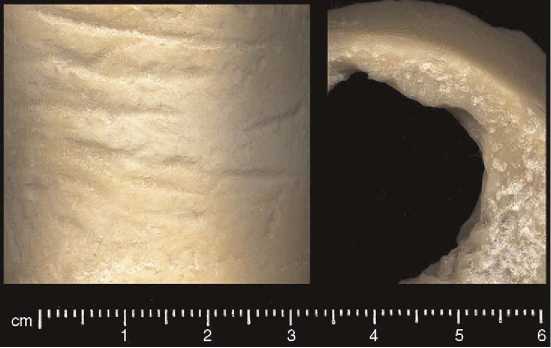
Figure 3 Gnawing marks from a medium-size carnivore (dog).
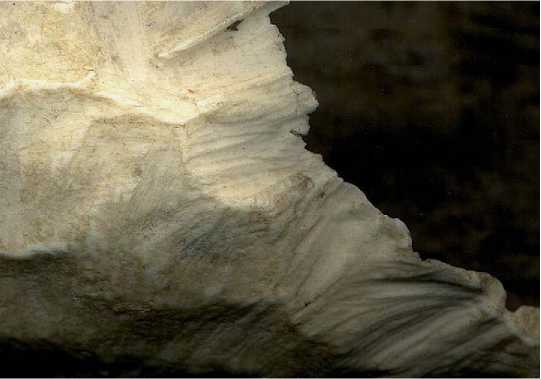
Figure 4 Gnawing marks made by a porcupine on a cow limb bone.
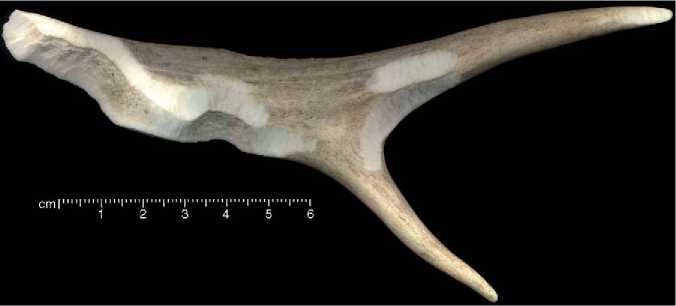
Figure 5 Gnawing marks made by small rodents on a segment of deer antler.
Of tool design and the distribution and types of microscopic textures or glosses on the artifact (see Lithics: Analysis, Use Wear).
Direct observation of the phenomena of interest is not possible in the historical disciplines of palaeontology, archaeology, sedimentary geology, and forensics. Cause instead is inferred from the remains and traces left by those phenomena. Causal agents may be identified from the diagnostic patterns of damage that they create, typical assemblages produced in recent contexts, or both. Signatures or patterns may not be unique to one type of agent, however. This is the interpretive dilemma of ‘equifinality’. The range of potential explanations for an observed case ideally is reduced through a stepwise process of hypothesis testing (a series of logically ordered, constrained ‘if-then’ conditions). Cross-referencing of distinct lines of evidence is essential for excluding the competing explanations. In the case of marine shells in an archaeological site, for example, a high incidence of burning damage coupled with consistently sharp break edges on the shells of large-bodied species might be expected if they represent waste from humans’ meals; wave-worn small shells with fresh perforations in the same site might instead suggest the use of the shells as ornamental material (Figure 9). Other (nontaphonomic) classes of evidence often are needed to fully eliminate or confirm each proposition. For example, if the shell material described above occurs only around hearths (fire places) in an archaeological site and never outside of the site perimeter, then noncultural explanations are very unlikely.
The order of events that contributed to assemblage formation can also be very informative. If two types of surface damage indicative of two distinct agents are encountered in an assemblage, overprinting of one type with another on bone specimens would, if consistently expressed, indicate the probable sequence of modification events. Some agents may be incompatible in the same space and time, such as a hibernating bear
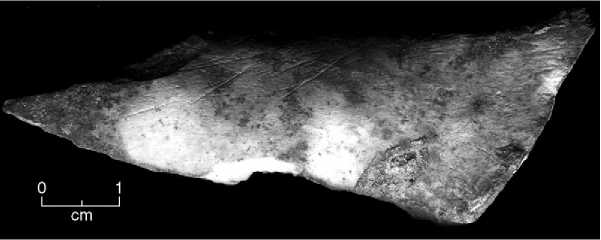
Figure 6 Cut marks from stone tools on deer bones from an Upper Palaeolithic site.
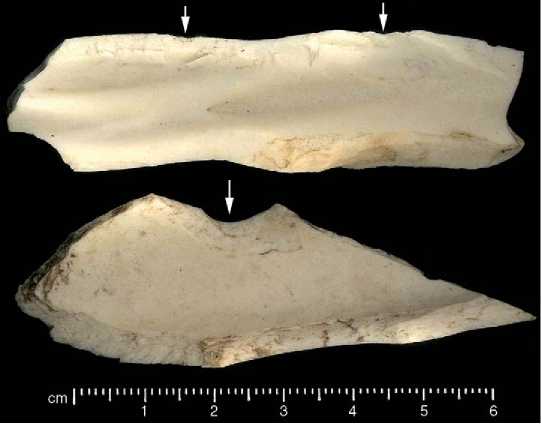
Figure 7 ‘Cone’ or conchoidal fractures (arrows indicate contact points) that may be produced by compression in the jaws of a large carnivore or, more commonly, by impact from a hammer (stone or metal) wielded by a human; cone fractures tend to be generated by consumers when breaking open large bones to obtain marrow; tool-induced cone fractures often display a roughened, shallow depression at the point of impact.
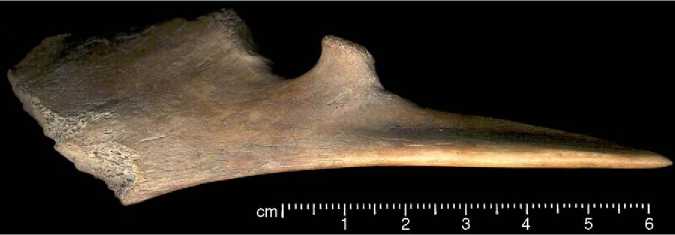
Figure 8 Polished surfaces caused by repeated use of a pointed bone tool (awl) designed for puncturing hides or basket-making.
Versus noisy humans and their fires in the same cave, or atmospheric weathering that requires exposure on the ground surface versus fossilization (mineral replacement) of the same bone following its burial in sediments. Evidence of incompatible agents in an assemblage of bones may therefore suggest that the material accumulated over an extended period of months, years or longer.
While damage to skeletal remains is a rich source of information about the history and causes of assemblage formation, it undermines the identifiability of the remains (Figure 10) at the taxonomic, individual, and anatomical levels. Losses in identifiability (attrition) can be disproportionate, or biased, because of the differential fragility of species’ skeletons and the heterogeneous structure of elements within the
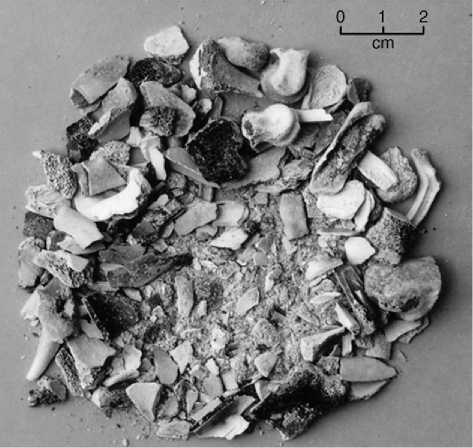
Figure 10 Burned mammal bones subjected to trampling; because high temperatures cause bone materials to become brittle, burning damage coupled with compaction may greatly reduce the identifiability of skeletal specimens.
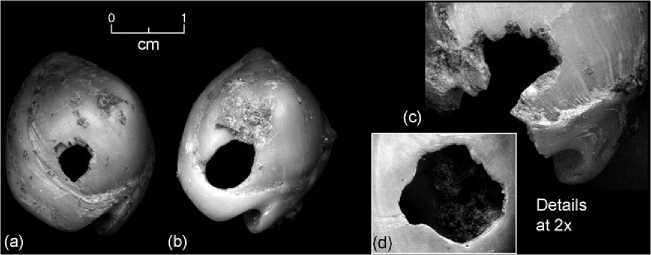
Figure 9 Examples of marine shells modified into ornaments by Upper Palaeolithic humans: (a), (b), perforated specimens, one wave worn and one in fresh condition, implying that at least some of the shells selected for ornament making were obtained from dead, beach-cast sources; (c), (d), details of holes made by humans using a punch tool, which resulted in accidental breakage of shell lip in one case (c) and scratches from punching tool near the perforation on another (d).
Skeleton. With time, these conditions often lead to significant biases in content relative to what was originally present in the accumulation. The issue of differential attrition was explored formally by C. K. Brain in the 1960s in a comprehensive study of fossil and recent bone assemblages in South Africa. The concept and the tools for its investigation were refined further in the 1980s onward by R. L. Lyman and others. Diverse causes of attrition in situ have been identified, ranging from ravaging and selective deletion by carnivorous animals, to high-energy water transport, weathering, and mineral dissolution. The first objective in studies of attrition is to determine what components of once-whole organisms or their skeletons are missing relative to a complete anatomical model. The second question may focus on why and
How the biases came about, using multiple lines of evidence and including superficial damage patterns.
Two distinct domains of attrition are now recognized, mechanical types described above and chemical types, and they differ in their potential biasing effects. The distribution of bone macrostructures in the skeleton bears a loose but predictable relation to resistance to mechanical forces of decomposition, less to the effects of chemical decomposition. Preservation conditions, and the biases introduced by differential preservation, can be predicted partly as a function of variations in skeletal macrostructure (e. g., differing porosity of compact and spongy bone, aka structural or bulk density), chemical stability of the material, or mineral density (the percentage of organic vs. inorganic constituents within the material). Alteration of materials can occur at any structural scale, from molecular to macrostructural. However, differential destruction of mammalian skeletons is most often considered in terms of the relative proportions of spongy (cancellous) versus compact bone parts, bones versus teeth, and immature versus mature bone, because of a historical (and often necessary) preoccupation with the biological identity of the materials under investigation.
Studies of chemical alteration, or ‘diagenesis’, have expanded greatly in recent years. This area of tapho-nomic research developed principally from discoveries in structural biology and applications for stable isotopic analysis, a technique that uses mass spectrometry to measure the different isotopic forms of low mass (light) elements such as oxygen, hydrogen, carbon, nitrogen, and sulfur in samples (see Stable Isotope Analysis). Diagenesis refers to molecular changes in skeletal materials postmortem, including alterations in chemical makeup, mineral crystallinity, porosity, and mineral dissolution and replacement. Chemical alterations of animal or plant remains are tied directly to sedimentary conditions, especially matrix pH as influenced by water and any kind of organic input that raises the acidity of that matrix.
Taphonomic methods have revolutionized scientific and lay perceptions of the nature and behavior of extinct organisms, including humans and human ancestors. Establishing the agents of bone collection and modification is prerequisite to dietary analyses in the archaeology. In zooarchaeology, taphonomic data provide critical insights about the carnivorous behavior of prehistoric humans and, in the Early Pleistocene, ecological competition between extinct members of the human evolutionary tree (taxonomic tribe Hominini of the family Hominidae) and large cats and hyenas. Widespread integration of tapho-nomic analyses in archaeological research is also responsible for the decline of ‘living floor’ or snapshot reconstructions of archaeological sites in favor of dynamic or cumulative models. Recent innovations in taphonomic methods offer numerous means for distinguishing activity areas within archaeological sites, selective transport of animal parts, and differential preservation in sediments, as well as more effective ways to investigate food-processing practices and their energetic consequences in prehistory and the recent past. Perhaps more than any other subset of archaeological research, taphonomic approaches have promoted a fundamental shift from static to dynamic conceptions about how archaeological and palaeontological records form.
See also: Archaeozoology; Behavioral Archaeology; Invertebrate Analysis; Lithics: Analysis, Use Wear; Phytolith Analysis; Pollen Analysis; Sites: Formation Processes; Stable Isotope Analysis; Vertebrate Analysis.