Optically stimulated luminescence (OSL) A method in luminescence dating using a narrow bandwidth of visible light to stimulate a luminescence signal in order to measure the accumulated radiation dose during the time elapsed since material containing crystalline minerals was either heated (lava, ceramics) or exposed to sunlight (sediments). radionuclide An atom with an unstable nucleus, which is a nucleus characterized by excess energy which is available to be imparted either to a newly created radiation particle within the nucleus, or else to an atomic electron. thermoluminescence (TL) A method in luminescence dating using heat to stimulate a luminescence signal in order to measure the accumulated radiation dose during the time elapsed since material containing crystalline minerals was either heated (lava, ceramics) or exposed to sunlight (sediments).
Luminescence is light emitted from crystalline materials after an initial absorption of energy. It is distinguished from similar phenomena such as fluorescence by a time interval between absorption and emission, a delay that allows, under ambient conditions, an accumulation of stored energy that can serve as a clock. Energy is accumulated by absorption of ionizing radiation, typically from natural radiation sources in the sample and its surroundings. Ionization produces free charge carriers (electrons and electron vacancies, or holes) that then become trapped at local, charge-deficient defects within the crystal lattice. Absorption of other energy, typically heat or light, may release electrons and holes from these traps, and their recombination with opposite charge as they return to ground state produces luminescence. Where light releases the trapped charge, the resulting signal is called optically stimulated luminescence (OSL). Where heat is the agent, the signal is called thermoluminescence (TL). Either can be used for dating.
Only defects that do not lose their trapped charge under ambient conditions can be used for dating. From these, the intensity of luminescence is proportional to the amount of trapped charge accumulated since the last significant exposure to heat or light. If the average radiation dose rate over time is known, this accumulated charge can be related to elapsed time. Because of long half-lives of the major natural radionuclides (238U, 232Th, and 40K), the dose rate is nearly constant over archaeological time, allowing current dose rates, in most cases, to be used to estimate average dose rate.
Figure 1 Is a simplified version of what happens. In remote geological time when the crystal structure is formed, all traps are empty. With time, the traps fill as a function of ionizing dose rate and availability of empty traps. If all traps fill, the sample is said to be saturated. When the sample is exposed to sufficient heat or light, the traps are emptied (the zeroing mechanism). After being emptied, the trapped charge builds up once again, a cycle that may be repeated several times, until the sample is retrieved for dating. The sample is heated or exposed to light in the laboratory and the resulting luminescence is measured. The intensity is calibrated against artificial irradiation in the laboratory to determine how much ionizing dose is required to replicate the natural signal. This is called equivalent dose (De) and dividing by the dose rate yields an age.
The event dated is the last sufficient exposure to heat or light. This allows dating of materials heated in antiquity, such as ceramics or burned lithics. Sediments exposed to light when deposited and subsequently buried can also be dated. The main sample requirement is a suitable dosimeter - a crystalline material that produces a luminescence signal and is sensitive to dose. Quartz and feldspar (particularly
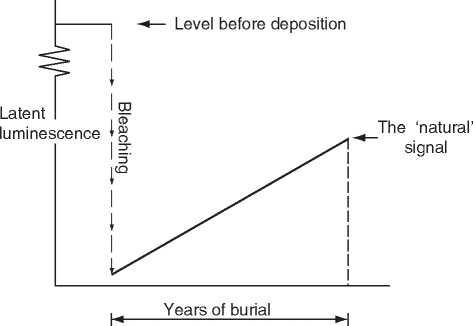
Deposition
Laboratory
Measurement
Figure 1 Simplified schematic of the process of luminescence dating (Aitkin 1998). Latent luminescence refers to the accumulation of trapped charge, while ‘bleaching’ can be from exposure to either light or heat.
Potassium feldspar) are the two most common dosimeters used. Their ubiquity gives luminescence a wide range of applicability, often being suitable where materials for other dating methods (see Dating Methods, Overview) are not present. Small surface scatters of artifacts, for example, have been dated by luminescence. But it does not work everywhere. A sensitive dosimeter may not be present (quartz often has a poor signal) or the trapped charge may ‘leak out’ over time (called anomalous fading and often a problem with feldspars if not corrected for).
Laboratory procedures involve measuring De and the dose rate. Most research has been directed at getting an accurate measure of De - making sure only stable traps are sampled, correcting for changes in luminescence sensitivity between natural and artificially irradiated aliquots, testing for the possibility the sample was only partially zeroed in antiquity, and taking into account anomalous fading. But there can also be problems getting an accurate assessment of dose rate, including lower efficiencies of alpha irradiation, complicated radioactive environments, changing dose rates through time, and uncertain moisture contents. These complications provide wide room for error, but if measurements are careful, the method is remarkably robust, and luminescence ages often compare favorably with independent dating results (Figure 2). Improved methods and instrumentation have allowed precisions of 5-10% to be achieved routinely. But every date, just as the case for any other dating method, needs to be evaluated critically, requiring archaeologists to understand the intricacies of the method.
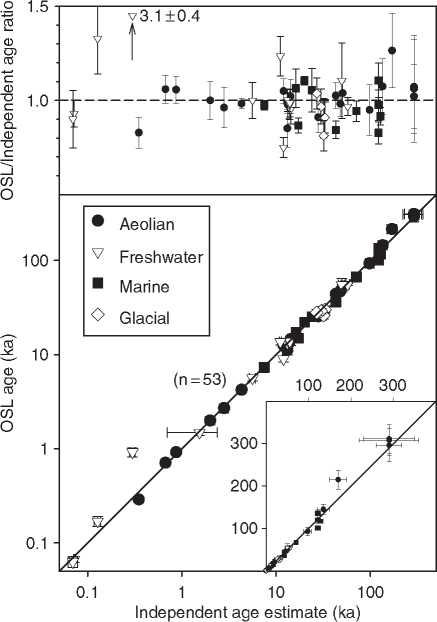
Figure 2 Comparison of quartz OSL ages with independent age estimates using a logarithmic scale (the inset shows the linear scale). The ratio of OSL to independent age is plotted in the top part of the figure, showing some statistical disagreements, although whether the OSL or independent ages are in error is not known. Data compiled by Murray AS and Olley JM (2002) Precision and accuracy in the optically stimulated luminescence dating of sedimentary quartz: A status review. Geochronometria 21: 1-16.
The upper limit on dating depends on the onset of saturation or on thermal fading of relatively shallow traps, which are deep enough for dating young samples but which lose some of their signal over long times. Saturation comes earlier in quartz than in feldspar, limiting dating by quartz to about the last 100000 or 200 000 years, depending on the dose rate, while feldspars, if anomalous fading can be controlled, may date up to 1 million years. That luminescence is applicable well beyond the 40000-year range of radiocarbon (see Carbon-14 Dating) has allowed significant applications in Old World archaeology. Dating burned lithics has refined the chronology of the Middle Palaeolithic and the transition to the Upper Palaeolithic in Europe and the Near East. Dating buried sediments has provided detail on the poorly known chronology of the Middle Stone Age in sub-Saharan Africa.
The lower limit on dating depends on the strength of the signal from low doses, but nineteenth and even twentieth century dates of good precision have been obtained. An advantage of luminescence for young
Samples is that it produces calendar ages that need no further calibration against independent dating evidence. Luminescence can often exceed the precision of radiocarbon for dates during the past 1000 years, when the calibration curve for radiocarbon often produces multiple intercepts.
Although luminescence often lacks the precision of radiocarbon or tree-ring dating, it has one distinct advantage that makes it the method of choice in some situations even where other datable materials are available. A distinction is often made between ‘dating’ events, the event actually addressed by the dating method, and ‘target’ events, the event of archaeological interest. Where the two do not coincide, bridging arguments are required to associate the two events. With luminescence, bridging arguments are often obviated because the dating event and target event are the same. Ceramics are dated to when they were last heated, usually when made or used, which is what archaeologists want to know. Lithics are most likely dated to when they were accidentally burned either during manufacture (normal heat treatment is usually not to high enough temperatures) or during occupation, again an archaeologically relevant event. For sediments, attempts to date them by their contents using methods like radiocarbon are often unsuccessful because deposition has incorporated old material, but luminescence dates the depositional event itself.
This advantage is well illustrated by attempts to tease apart mixed assemblages. Ceramics of different ages found in the same deposit cannot be easily differentiated by, for example, associated charcoal using radiocarbon. But luminescence has demonstrated that ceramics found together, even side by side in the same pit, can be separated in age by centuries. Here the high precision of radiocarbon must be traded for the better accuracy of luminescence. Dating individual artifacts by luminescence also allows measurement of the tempo of technological change. Artifacts successions are usually dated in relative terms by averaging methods like seriation or ceramic crossdating, which can detect changing modes, but not beginnings and ends.
Most current research in luminescence is directed at dating unconsolidated sediments, not only because of wide applications in quaternary geology, but because, unlike ceramics or lithics, all grains in sediments may not be the same age, either because some were not fully reset at the time of deposition or because of mixture after deposition.
This problem has been addressed by either component analysis of the luminescence signal or by measuring single grains. The luminescence signal is a composite of signals arising from traps which differ in their response to sunlight. Various techniques have been devised to isolate the ‘fastest bleaching’ component which is most likely to have been reset to zero at the time of deposition. Comparison with a slower bleaching component informs on the extent of bleaching. By determining De on single grains, or single aliquots containing a small number of grains, the fully reset grains may be isolated from the partially bleached ones and mixed assemblages may be identified. Measuring single grains has become practical by advances in instrumentation and in single-aliquot methods for determining De, although interpreting the distribution of De values from grains of a single sample is not always straightforward.
Identifying mixtures is important for understanding site-formation processes and assessing the integrity of stratigraphy. These problems are particularly relevant for controversial issues such as colonization of Australia and settlement of the New World, both areas where luminescence dating has been applied. Isolating only well-bleached grains has also proven useful in dating construction episodes in monumental architecture and in dating the covering of rock art by mud wasp nests.
Seealso: Amino Acid Racemization Dating; Carbon-14 Dating; Dating Methods, Overview; Electron Spin Resonance Dating; Obsidian Hydration Dating.