Caves and rockshelters are subject to a number of depositional and postdepositional processes that not only preserve the archaeological record, but can also significantly modify it and how we interpret it.
Depositional processes involve the accumulation of coarse deposits (pebble-size and larger) and finegrained materials (sand, silt, and clay) from both within and outside the cave or rockshelter. Coarse material (eboulis, in French) is normally a product of roof fall or wall collapse, and results from the dissolution of the bedrock along joints, the breakdown of the bedrock by freeze-thaw processes, or tectonic activity. The Eboulis can vary from centimeter-sized clasts to blocks over a meter in diameter.
The finer fraction in cave and rockshelter deposits can come from a variety of sources. These include the following:
1. Infiltration of reworked soil material through joints or solution cavities in the bedrock. This material accumulates along the walls and on the floor of the cave or rockshelter as small mounds, which can be re-mobilized by flowing water or human activity, such as trampling.
2. Fluvial deposition is also one of the ways in which caves and rockshelters in stream valleys may receive alluvium. When streams are adjacent to or very near valley walls, coarse-grained sediment, including sand and gravel, may be delivered to rock-shelters and cave mouths through lateral accretion of point bars. In contrast, fine-grained alluvium (mostly silt and clay) held in suspension may be deposited during floods, a process known as vertical accretion. Rodgers Shelter in the western Ozark Highland of Missouri is a good example of a rockshelter containing a sequence of lateral and vertical-accretion deposits. This archaeologically rich shelter remained at or only a few meters above the elevation of the floodplain of the Pomme de Terre River throughout the Holocene.
Alluvium also occurs in karstic caves. For example, at Sheriden Cave, a Clovis habitation site in Ohio, finegrained sediments display evidence of alluvial transport. In general, coarser sediments, including sand and fine gravel, were deposited near the cave entrance, and silt and clay were deposited in laminar layers in the lowest passages. This depositional sequence is similar to the one described for Ohio Caverns.
3. Eolian accumulation, which takes the form of sand deposition associated with coastal dunes and eolianites (fossil dunes) is another way. Many important caves and rockshelters have eolian sand accumulations that are tied to worldwide fluctuations in sea level (e. g., Tabun Cave, Israel; Gorham’s and Vanguard Caves, Gibraltar; Blombos and Die Kelders Caves, South Africa; Akrotiri Aetokremnos Rock-shelter, Cyprus). Build-up of finer, silt-sized material in caves and rockshelters is volumetrically less important but is clearly visible; it is generally linked to eolian dust deposition or reworking of eolian silts (e. g., Dust Cave, Alabama).
4. In addition to coastal eolian deposits, finegrained marine littoral deposits occur as sandy beach sediments in caves and rockshelters (e. g., Yarimburgaz Cave, Turkey). Coarse boulders representing former high-energy shorelines also occur (e. g., Southeast Alaska sea caves).
5. Slope deposits are another source. There are two general types of slope deposits: colluvium and slope wash. Colluvium is poorly sorted deposits of sediment transported chiefly by gravity. Slope wash is laminated, well-sorted deposits of soil and sediment that has been moved down a slope predominantly by the action of gravity assisted by water in the form of sheet flow or concentrated flow in rills. Slope deposits may form a berm or talus cone in front of a rock-shelter or cave entrance. From there, they can be washed back into the shelter or cave by runoff or high-density mudflows (Figure 5).
6. Attrition is also one of the sources. Solution weathering, including hydration and carbonation, often causes grain-by-grain disintegration of sandstone and bioclastic limestone, which produces a steady rain of sediment to the floor of a cave or rockshelter. This process is a major source of sediment in several important rockshelters, including Akrotiri Aetokremnos in Cyprus and Meadowcroft in Pennsylvania.
7. A major aspect of the sediments, and one that has received surprisingly little attention, is their anthropogenic nature. These human-derived materials are obvious and widespread in Holocene sites around the Mediterranean. However, significant human inputs are also noteworthy from Palaeolithic and Middle Stone Age (MSA) sites in the Old World and Africa (Figures 5 and 6a). Most typical are midden-like accumulations of bone and lithic debris, but remains of combustion features - both in situ and reworked (e. g., hearth rake-out and dumping of ashes and charcoal) - are increasingly being recognized and understood. In certain instances, such as Kebara and Hayonim Caves, anthropogenic accumulations constitute the majority of the depositional fill and substantially exceed geogenic contributions.
8. Finally, biogenic additions are also very common and subtle. Bat and bird guano are common in caves and rockshelters today, but in Pleistocene deposits it is difficult to detect them directly in the field. However, guano can be documented by microscopic and other analytical evidence (e. g., X-ray diffraction (XRD), Fourier Transform Infrared Spectrometry (FTIR)), in the form of phosphate or other minerals which generally are a product of a series of mineralogical transformations induced by the original guano.
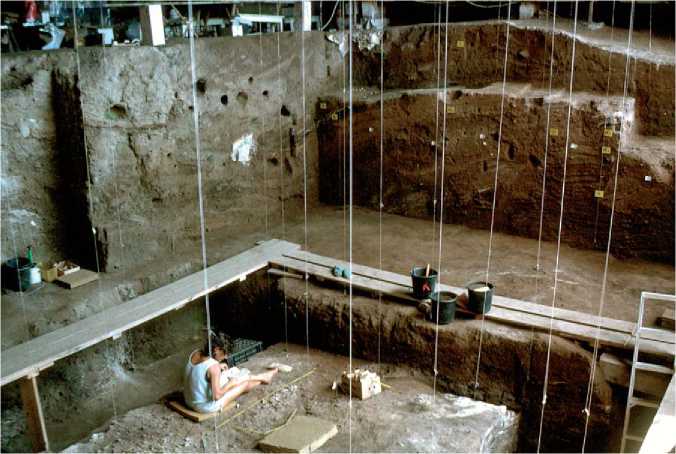
Figure 5 Kebara Cave looking toward the southeast corner. Note the exposed south wall on the right, in which the mostly Upper Palaeolithic deposits are dipping from the entrance of the cave (right) to the back (left). This bedded silt - and sand-sized material is in large part composed of Middle Palaeolithic combustion features that have been reworked by sheetwash from the entrance and transported toward the back. This dip initially resulted from a large-scale subsidence event into a subsurface swallow hole that took place near the rear of the cave during late Middle Palaeolithic times.
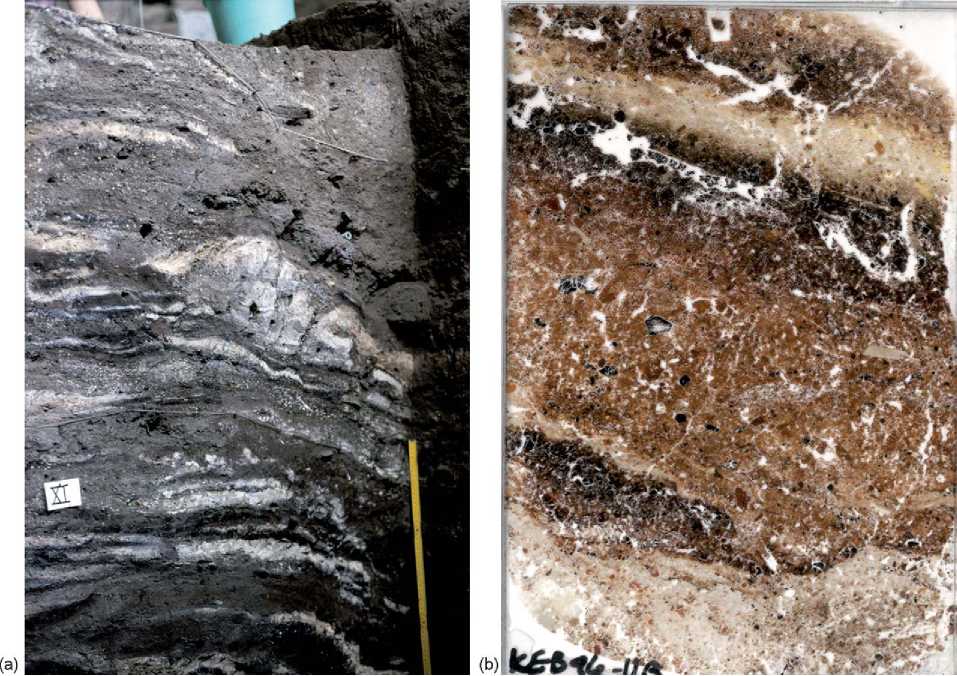
Figure 6 (a) A sequence of combustion features and anthropogenic deposits from the Middle Palaeolithic layers at Kebara Cave, Israel. The whitish zones were originally calcareous ash accumulations, which have been subsequently altered by diagenesis to apatite (calcium phosphate) or other phosphate minerals. At the right, the stepped-like and offset nature of the ashes is a product of subsidence of the underlying deposits into subsurface depressions. The ruler is 50 cm long. (b) Macrophotograph of sequence of thin combustion zones from the Middle Palaeolithic layers of Kebara Cave (cf. part (a)). Note the sequence of a dark charcoal-rich layer overlaid by lighter ashes. Scale: length of photograph is 75 mm.
Postdepositional processes can affect the archaeological record in caves and rockshelters in a number of ways, and generally fall under the term, ‘diagenesis’. Physical modifications include displacements of bones, lithics, and anthropogenic debris by many of the depositional processes cited above (e. g., mudflow, sheetwash) (Figure 5). Erosion by wind (‘deflation’) and water (e. g., streams, storm-generated waves along coasts) can totally remove previous accumulations. Similarly, colluviation can transport deposits near the site entrance to further back in the cave or rockshelter, removing both artifacts/ecofacts, and dismantling previously formed features. In addition, reorganization of cave deposits through subsurface slumping is a typical process in karstic settings, and can take place even when the surface deposits are dry and somewhat consolidated.
The effects of chemical modifications can be more subtle but no less important. The most striking and visible is the precipitation of calcium carbonate that takes the form of crusts and layers (‘flowstone’, ‘travertine’) (Figure 7), stalagmites, and cemented detritus colloquially known as ‘breccia’. Flowstones and travertines, when pure, are very valuable for two reasons. Firstly, they can be dated by uranium-series dating techniques, thus enabling the construction of temporal frameworks up to 350 000 years, well beyond the limits of radiocarbon. Secondly, they furnish detailed climatic records as expressed by oxygen isotope contents of individual calcite layers.
On the other hand, the presence of guano, organic acids, and acidic dripping waters can result in the partial or even total dissolution of carbonate and the formation of other minerals, such as gypsum. In some instances, a marked reduction of volume of the original deposits can occur (up to 50%), such as Die Kelders Cave (South Africa) Furthermore, in cases of extreme diagenesis, major mineralogical transformations can take place involving the formation of various phosphate minerals (Figure 6a), the breakdown of clays, and the precipitation (neoformation) of silica. The ramifications of such transformations involve the complete destruction of bone from all or parts of the cave or rockshelter, as well as the close monitoring of dosimetry measurements since radioactive isotopes can be concentrated during diagenesis. These ‘hot spots’ must be taken into account when dating techniques such as thermoluminescence (TL), electron spin resonance (ESR), or optically stimulated luminescence (OSL) are used. Awareness of such diagenetic changes, therefore, allows for more accurate dating of deposits, and also controls on the spatial distribution of bone across a site, which may not
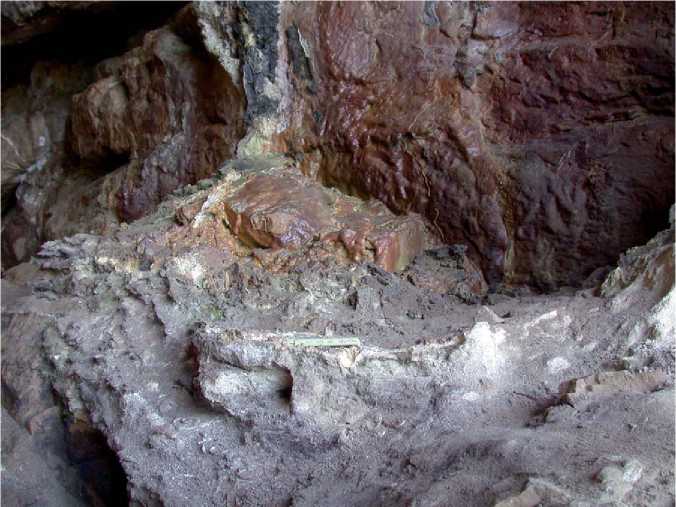
Figure 7 Secondary calcium carbonate draping over wall and MSA deposits at Cave 13b from Pinnacle Point, South Africa. The 20 cm ruler rests upon a thin whitish crust (flowstone), which overlies sandy deposits that were blown into the cave. In turn, these sands cap a series of darker sediments containing combustion features that are rich in burned shellfish. These deposits are currently being studied, and uranium-series dating of the flowstone combined with OSL dating of the underlying sand should provide independent dating checks and tight chronometric control of the infilling of the cave and associated occupations.
Relate solely to human activities but postdepositional, bio-geo-chemical ones.
Sedimentological studies of cave and rockshelter deposits have advanced markedly in recent years. Up to the end of the 1970s, researchers tended to use a somewhat standardized set of analytical techniques. These consisted of grain-size analysis, morphology of clasts and grains, analysis of heavy minerals (those with high specific gravities), and other mineralogical analysis using X-ray diffraction. A number of chemical analyses were also employed, measuring amounts of organic matter, calcium carbonate, and phosphate. These analyses were carried out on bulk samples collected from main stratigraphic units.
More recent research has made use of soil micromorphology, the study of intact blocks of sediments under the petrographic microscope. With this technique, undisturbed material (Figure 8) is embedded in polyester or epoxy resin, sliced and mounted on a glass slide, and finally ground to a thickness of 30 pm (Figure 6b). The use of these thin sections enables the researcher to observe mineralogical composition, size and shape of the particles, and the finer matrix. More importantly, because the original geometry of the components is preserved, it is possible to isolate depositional effects from postdepositional ones. Thus, original calcite from limestone eiboulis can be distinguished from secondarily precipitated carbonate. Micromorphology has been effectively applied to many cave and rockshelter sediments that focus on important archaeological issues. These topics range from site formation processes in Palaeo-Indian sites and the early appearance of humans in the New World, to the use of fire in Zhoukoudian, China and in the Middle Palaeolithic.
Optical microscopy has its limits, however, and more sophisticated analytical techniques are now more commonly employed, particularly in the study of diagenesis. FTIR and electron microprobe analysis have been invaluable tools to evaluate the type and extent of diagenesis. Such studies have raised our awareness of these processes, which are crucial to a proper understanding of the archaeological record and how it has changed through time.
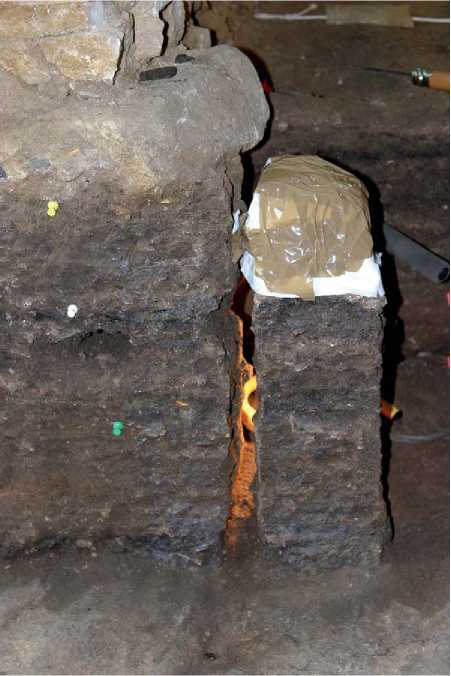
Figure 8 Middle Palaeolithic cave site of Pech de I’Aze IV, Dordogne, France. Shown here is an in tact block of sediment being removed for micromorphological analysis from organic-rich sands with remains of combustion features shown by the dark bands. Length of column is c. 30 cm.
And postdepositional processes, coupled with more sophisticated analytical techniques than existed even a decade or so ago, provide clearer windows into the activities, subsistence strategies, and behaviors of former inhabitants of cave and rockshelter sites.
See also: Electron Spin Resonance Dating; Luminescence Dating; Rock Art; Seasonality of Site Occupation; Soils and Archaeology.