There is an abundance of research published on fossil bone taphonomy, one focus of which is the determination of whether fossil bone accumulations were made by Plio-Pleistocene hominids or carnivores. This literature has been succinctly summarized by Travis R. Pickering (2002) in his review of criteria that have been proposed to accomplish this differentiating purpose. Pickering discusses seven criteria that have been proposed by various workers (Cruz-Uribe 1991, in particular), and suggests that only three are really up to the task: (1) carnivore-ungulate MNI ratios (carnivore assemblages have more carnivore remains); (2) intactness of limb bones (relatively high frequency of long bone mid-shaft survival in carnivore assemblages); and (3) bone surface modifications (chewing marks in carnivore assemblages). In the assemblages we studied, species identification was very difficult due mainly to small fragment size, so we are hesitant to employ our crude ungulate-carnivore minimum number of individuals (MNI) ratios for any sort of even semi-rigorous analysis. However, our assemblages were well suited for the second and third criteria. In light of our forensic orientation, we could identify many more perimortem taphonomic features, as the reader will see in the following listing of what we looked for. Unfortunately, our examinations could rarely identify scavenged bones where teeth and/or tool marks were evident (Selvaggio 1994). As will be discussed in various assemblage descriptions, we assume that scavenged bone was abundant when a hyena presence could be recognized. Taphonomic research is also aided by actualistic study, as illustrated in Figs. 2.5-2.8.
1 Provenience. Most of the assemblages were excavated by archaeologists and paleontologists using horizontal and stratigraphic units, such as squares, levels, layers, and distance fTom some monument or datum point. However, much of that information was not kept with most of the faunal collections; that is, point provenience was lacking for nearly all small pieces. While we have tried wherever possible to obtain this precise point of origin information, we have been far from successful. But this deficency is
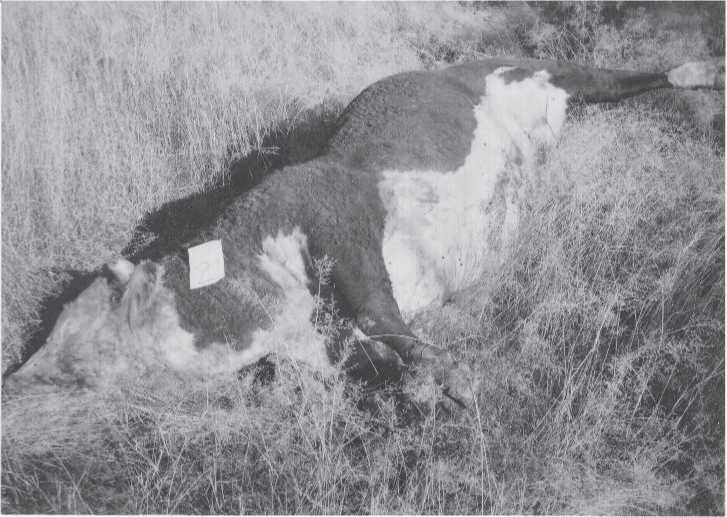
Fig. 2.5 A large dead animal, time sequence 1. First in a series to illustrate natural surface diagenesis of a large beef bull. Day 2 after death. Bloating has begun. Flies are abundant. No scavengers yet. November 7, 1988. Relatively isolated Upper Sonoran habitat on southeastern Arizona cattle ranch (Kali T. Holtschlag color Adams Ranch 11-7-88:0).
Probably not important because wherever we have been able to check for significant stratigraphic and temporal differences in our perimortem damage variables - the ones we are mainly concerned about - none was found. We have generally been unable to test for significant stratigraphic differences in species content due to the limited number of bone pieces with specific identifications. Moreover, where we do have stratigraphically controlled sub-samples, there are rarely enough pieces in each unit to give much statistical confidence, even in simple two by two chi-square comparisons. Hence, all things considered, both good and bad, we present our observations on a pooled intra-site basis. In a sense, then, we are presenting our assemblages as representative of a generalized late Pleistocene time except for the Primorsky Territorial site of Boisman II and the Yenisei River site of Yelenev Cave, both of Holocene time. We follow Kuzmin and associates for late Siberian Pleistocene carbon-14 dates (Kuzmin and Orlova 1998, Vasili’ev et al. 2002).
2 Species. Whole or nearly whole skulls, and many types of teeth are, with experience, easy to identify (examples shown in Figs. 2.9-2.10). Many isolated mammalian whole bones and even fragmentary pieces with only one anatomical end, such as the distal or proximal end of a femur, can also usually be identified to at least the genus level, and often to the species level. Most of the genus or species identifications in this book were made or confirmed by Ovodov. Ovodov is very conservative in his identification of
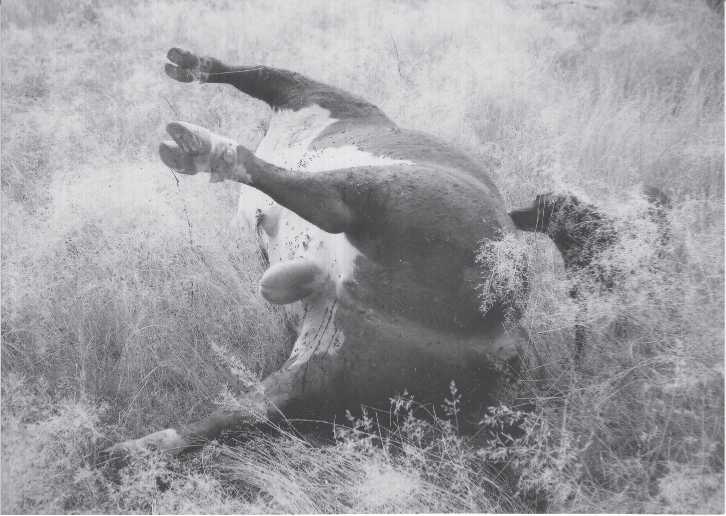
Fig. 2.6 A large dead animal, time sequence 2. Bloating pronounced. Limbs raised because of internal gas. Flies abundant. Undated but film fTame number near to Fig. 2.5. Odor pronounced. Tomsk mammoth was probably exploited for food before this stage of decomposition, or afterwards if only bone and oil were sought (Kali T. Holtschlag color Adams Ranch undated:4).
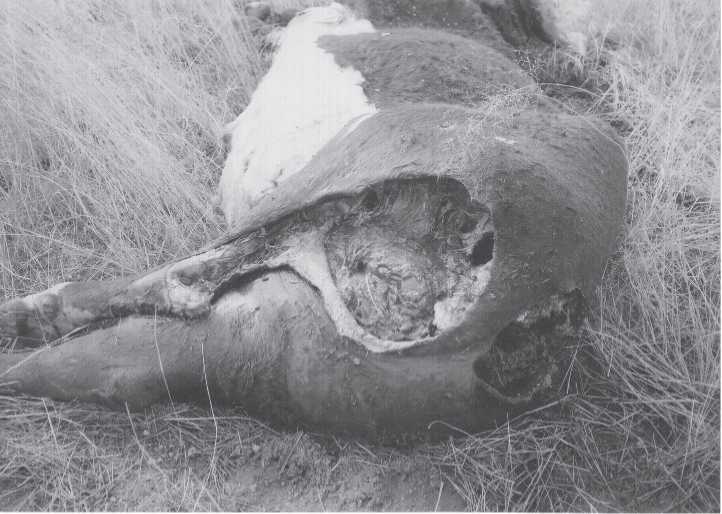
Fig. 2.7 A large dead animal, time sequence 4. Most gas has escaped. Scavengers have chewed into
Carcass from anal region. Tail is gone. Flies abundant (Kali T. Holtschlag color Adams Ranch undated:28).
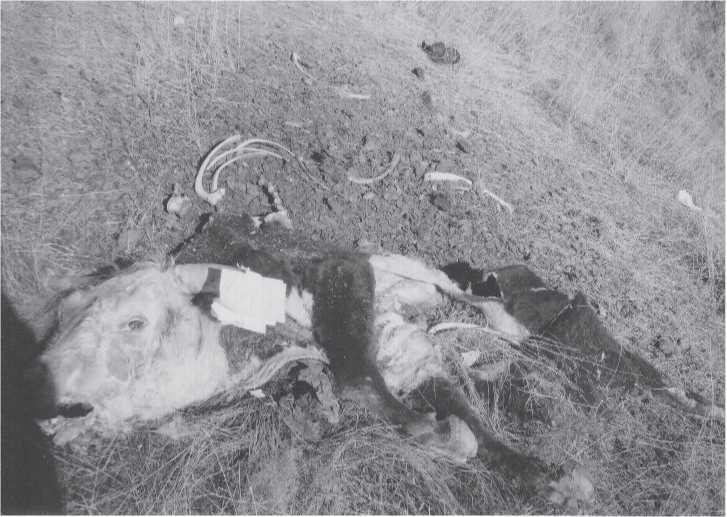
Fig. 2.8 A large dead animal, time sequence 7. About two months after death, scavengers have scattered ribs, other elements, and intestinal contents. Head is still attached to cervical vertebrae. About half of the animal is missing. Large scavengers in this southeastern Arizona high desert area include turkey vultures, crows, coyotes, and wild dogs. Many smaller animals, including birds, are known to scavenge also (Kali T. Holtschlag color Adams Ranch 1-19-89:32).
Species or genus, and even many relatively large pieces he has declined to classify. However, not all the unknowns are attributable to Ovodov, since he did not participate in the study of all assemblages. Many of the unknowns were scored as such by Turner. Our study largely excludes insectivores, bats, rodents, and birds. The rare exceptions are pieces with human processing marks.
If too much anatomical information is missing from a broken piece of bone to make genus identification possible, the piece may still possess enough morphology and size information so that it can be classified as a herbivore or carnivore, or even less definite, as a small-, medium-, or large-sized animal. We consider terrestrial animals such as fox, marten, marmot, and various hares to be small; deer, wolf, and boar to be middle-sized; and horse, bison, rhinoceros, and mammoth to be large animals. Elk and hyenas would be in the upper end of the middle-sized range, or in the lower end of the large-sized range. M. G. L. Mills (1990:11) gives average sizes for Kalahari brown and spotted hyenas. The latter are larger, males averaging 59.0 kg (130 lb) while females average 70.0 kg (156 lb). The Siberian cave hyenas may have been even larger than their African cousins if their average mass corresponded with Bergmann’s rule (mammalian species that live in colder environments are frequently larger than similar species living in warmer habitats). Bunn (1991:442) envisions small animals to be less than 50 lb (Thompson’s gazelle), medium-sized to be
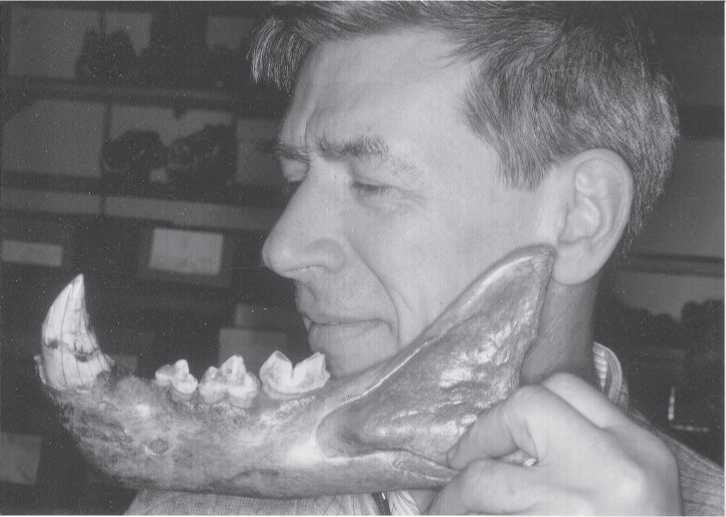
Fig. 2.9 Cave lion mandible. Nicolai Ovodov holds a cave lion jaw found in a cave near Irkutsk to
Show its relative size with that of a human. Dental morphology of lions is distinctive and easily identified (CGT color IHPP 6-4-87:27).
50-250 lb (impala), large to be 250-750 lb (zebra), very large to be 750-2000 lb (buffalo), larger animals to be >2000 lb (giraffe), and the largest to be more than 6000 lb (elephant).
In the maritime context of our Primorsky Territorial sites, sea otters would be small, various seals would be middle-sized, and walrus and whales would be large. With the exception of many teeth, broken pieces of long bone smaller than 10 cm in length are rarely identifiable, even to our size categories. The majority of broken vertebrae and ribs cannot be identified generically, but do fit our threefold animal-size categories reasonably well.
In this study we have paid relatively little attention to species identification for two reasons: (1) An overwhelmingly large number of recovered bone pieces could not be identified. In establishing our 2.5 cm minimal size criterion we found early on that almost no bone fragments smaller than 2.5 cm could be identified by Ovodov. Those that could be provisionally identified were almost always anatomically distinctive skull pieces. (2) We expected size biasing caused by preservation differentials (big bones preserve better), and human hunting practices. As David R. Yesner (2001:1) has made clear in his studies of ancient Alaskans: “Northern hunters generally focus on large game, whenever they can, especially those (like caribou) that aggregate in large numbers, both for reasons of availability and energetic efficiency.”
3 Skeletal element. There are a number of excellent guides to the skeletal anatomy of animals commonly found in archaeological contexts (Davis 1987, O’Connor 2000, and several others). Experience with one mammalian species is usually enough to make accurate
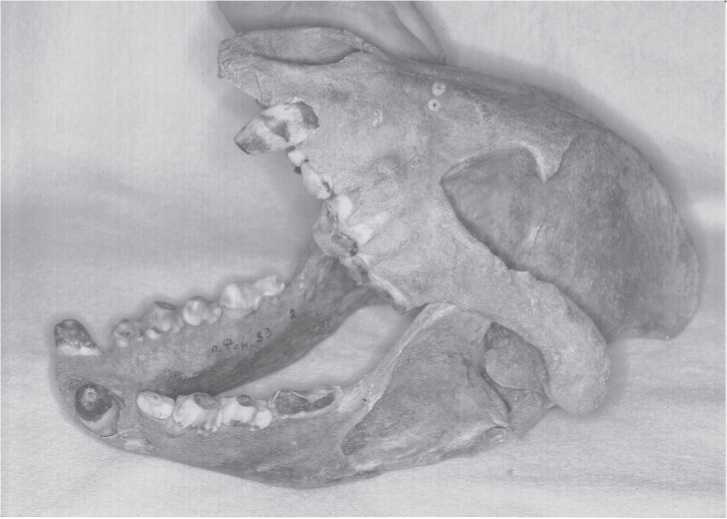
Fig. 2.10 Cave hyena skull belonging to a nearly complete old adult hyena skeleton found in Fanatic’s Cave, Khakasia, 1977. It was part of a paleontological assemblage found on the floor at the bottom of a vertical limestone shaft. The remains are estimated to be 30 000-40 000 years old. The animal was large, some 90 cm in height at the shoulder (Ageeva etal. 1978). As with the cave lion, cave hyenas are easily identified by teeth and cranial elements. This and other middle Yenisei river basin specimens means that there was likely no cis - or trans-Baikal gap in the longitudinal spread of these creatures (CGT color IHPP 6-4-87:28).
Whole-bone skeletal element identifications for many other species. For example, femurs of terrestrial mammals all possess similar biomechanical anatomy - ball and socket proximal joint, heavy slightly curved shaft, and rounded double surface distal joint. Whole vertebrae, crania, ribs, metapodials, and many other elements are too distinctive to ever be confused with one another. Teeth and bones can always be distinguished from one another. However, with breakage and fragmentation, reliable identification rapidly goes downhill. Reliability is usually high if a broken bone has an articular end or a distinctive cross-section, such as those of oval-shaped ribs, metapodial fusion vestige, and complex surfaces of cranial bones. Crania possess some of the most distinctive and easy to identify elements of the entire skeleton. For this reason, when the frequency of cranial elements is low in an assemblage, the odds are very good that some sort of segregation took place in the past. Hunters may have removed the heads and did not carry them back to their camp; the heads might have received special ritual placement away fTom the camp; or the heads or parts of heads were subsequently carried off by scavengers. Bunn (1991:444) remarked that skeletal elements are potentially helpful in identifying whether an archaeological site is a camp or kill site, or had some other function. As most of our assemblages came from caves, concern over site
Function is of less interest to us because all cave bone had to be carried in. Cave sites are not kill sites. Of more concern to us is who or what carried the bone into the caves.
4 Age. Because of the high degree of fragmentation in our assemblages, we limited age identification to adult, sub-adult, adult?, and unknown classes. While we very much wanted to have a highly refined set of age categories for considerations of seasonality, prey preference, MNI estimates, and other topics, such information was simply not contained in the remains we had to work with. In fact, simple age identification of adult and sub-adult was possible in only 70% of our total. Adult age was assigned when an epiphysis was fully fused to its diaphysis, when teeth were worn well into the dentine, when cortex bone was thick and dense, and when there was no surface indication of bone growth remodeling. Sub-adults would show the opposite of these mature conditions, i. e., incomplete epiphysial union, little or no crown wear, thin cortex bone relative to the amount of spongy bone, and the presence of porous growth remodeling areas. As with most of our perimortem taphonomy variables, there is a substantial inverse relationship with identification and piece size.
5 Completeness. There are several ways to assess and describe element reduction. Our simple three-category classification of whole, one anatomical end, and no anatomical ends, was created to help identify carnivore activity. These are illustrated in several of the sites discussed in Chapter 3. Many other workers have also observed that carnivores more often chew off the relatively weak ends of bones in their effort to extract marrow grease, rather than attack the harder mid-shaft regions. Humans, with the same objective in mind, more often smash the shafts of long bones with stone or bone/antler hammers and anvils, many times leaving the ends intact. However, the greater the degree of fragmentation, the more remote becomes the relationship between carnivores and completeness. Hence, the ease and reliability of observing this variable is outweighed to some extent by its containing causal information that differs from the initial and end phases of skeletal element destruction. Nevertheless, this variable is especially useful when combined with other indicators of carnivore or human processing activity.
6 Maximum size. This variable deals with the maximum dimension of a bone or piece, be it width, length or a combination of both. It is not a measure of anatomical length such as zygomatic breadth, cranial length, or crown-root height. Among its many uses are parametric tests for significant differences in bone reduction by carnivores and humans, and differences in archaeological assemblages fTom different localities. It is especially valuable in the analysis of pieces that have been digested by hyenas. We measured maximum size to the nearest millimeter using a 15 cm ruler or dial calipers for small pieces, and a measuring tape for pieces larger than 15 cm. Note that the size ranges in Table A1.6 are rarely lower than 2.5 cm. This is because the minimum size used in this study was set at 2.5 cm. There were thousands of pieces smaller than 2.5 cm in our various assemblages. Most had very little perimortem damage information other than color and breakage. Occasionally a smaller piece was scored for all 26 variables because it had one or more special qualities that we wanted to keep track of. Note also that there are no pieces larger than 82.5 cm. This is also partly our doing, since we did not consider whole bones for this study if they lacked perimortem damage. Keep in mind the objective of this work. It is not a study of anatomy or species, or traditional taphonomy. It is a more experimental study of perimortem damage.

Fig. 2.11 Damage types. The form of bone damage corresponds with skeletal element. Left to right: Top row: two maxillary fragments, tooth-bearing maxilla with teeth, two cranial pieces. Middle row: 11 splinters (horizontal), five flakes (vertical), one butt. Bottom row: one vertebra spine, one vertebra body, four ribs, one segment. Not shown are cracked open phalanx, and irregular shaped fragments (pelvis, scapula). Specimens mainly from Razboinich’ya Cave (CGT neg. IAE 7-6-00:1).
7 Damage type/shape (Fig. 2.11). In the initial stage of this investigation we wanted to create an original descriptive typology for the shape or form of a broken piece, something along the lines of classifications used in stone tool manufacturing, i. e., cores, flakes, blades, etc. Terms like “spiral fracture” or “blown-out tooth socket” are valuable for identifying conditions, but they do not identify shapes. Try as we might, we were unable to develop a set of shapes that was not so generalized as to be useless. Binford’s (1981) breakage classification did not fit well with the damage we were observing. Moreover, no classification that we dreamed up applied to all skeletal elements because the form of a broken piece is very much dependent on which bone it comes from. Cranial pieces break up into irregular shapes very different from those of ribs. The long bones, including metapodials, can splinter and flake, but rarely wind up in the chunky forms common to vertebrae. Phalanges when broken open look for all the world like cigarette butts, a shape rarely seen in other broken skeletal elements, etc. Thus, the terminology used in Table A1.7 is basically anatomical and element dependent.
Four damage categories include more than one skeletal or anatomical unit: (1) Long bone fragments, flakes, and splinters. We include many metapodial pieces here as well as any other skeletal elements broken into these shapes. (2) Undamaged. Any skeletal element can be placed in this class. Undamaged is basically elements that have only a few tooth dints or tooth scratches, or one or two cut marks. Minor damage of minimal extent, if any, defines this class. (3) Irregular. Odd-shaped pieces of pelvis, scapula, cranium, and unidentifiable are the main components of this category. (4) Mostly whole. Any element that is approximately 90% complete is placed in this group. Although we are far from satisfied with our damage shape classification, it turns out to be a useful adjunctive descriptive tool for characterizing overall perimortem damage. We would have been even more dissatisfied had we not developed some manner of shape and form damage classification.
8 Color. We initially tried to score color by using various color standards such as soil and paint reference scales. These standards soon proved to be needlessly precise. A single piece of bone might have six or even seven slightly different shades of color, the recording of which was time consuming, much too exact for our needs, and decidedly prone to intra-observer judgment variation. Judgment was plainly influenced by differences in institutional lighting, quality of bone cleaning, and end-of-the-day observer eye fatigue. Super precision was senseless because all bone is the same color before it acquires stain or is burned. We eventually settled on four categories that can be easily identified: ivory (unstained, creamy pale yellow to light brown due to washing and archaeological processing); white (like chalk); brown (moderately to heavily stained or charred); and black (burned or manganese-stained). Unstained ivory color characterizes cave bones. White is found in bone left in the open to become sun-bleached and heavily weathered. White can also occur with calcining, that is, severe burning. Calcined bone can be scratched very easily with one’s fingernail. Brown characterizes bone color fTom most open sites where staining is caused by mineral-bearing groundwater contamination. Rarely is the staining much more than surficial, as shown in scratches resulting from excavation. When a piece is colored brown throughout its cross-section, we have usually found the piece to have been scorched by fire. Black bone is considered stained when the color is surficial. This is usually in the form of “manganese flowers,” which are surprisingly common on bones from cave sites (see examples in various figures). Black bone is considered burned when the color is present throughout the cross-section, that is, the piece is carbonized or charcoaled. Burned black bone rarely holds a polish, whereas bone stained black usually has end-polishing. Only in one site, Okladnikov Cave, did we have difficulty deciding whether a few pieces were brown or black. These we scored as black brown. Burning is very important taphonomic information for identifying human presence in a site. But since burning is almost always recognized, at least initially, by color, we delegated burning to our color classification, rather than setting it up as another variable classification. Said another way, all black pieces are black in color, but not all black pieces are necessarily burned.
9 Preservation (see examples in various site assemblages). Bone preservation can be easily classified as good or poor, that is, ivory or chalky in hardness. Ivory-quality bone cannot be scratched with one’s fingernail, whereas chalky bone is readily scratched. Bone preservation is generally good in cave sites and poor in open sites. Special conditions such as permafrost or high calcium carbonate content of midden in open sites can enhance the quality of preservation. Chalky bone indicates that one or several destructive processes have acted on the bone. These processes range from seasonal dehydration and wetting, sunlight, weathering, thermal stress, denaturing, and so forth. We found that in a few sites some pieces of bone were both ivory and chalky. These were classified as intermediate.
10 Perimortem breakage (see examples in various site assemblages). Breakage may occur at any time in the life and death history of a bone. A fracture that exhibits bone reaction in the form of healing or infection is a life history event, an antemortem break. A fracture that has no sign of repair or inflammation, but does have a clean sharp surface that is smooth like broken glass and sometimes spiraled around the long axis of the broken bone, is a perimortem break. That is, a break that occurred at or around the time of death (or later in some circumstances). There are various forms of perimortem breakage, including embedded fragments (caused by a tooth puncture or hammer stone impact), circular conchoidal breakage (caused by bullet - or bat-like trauma), radiating skull fractures that originate at the point of weapon impact, and many other forms. All share the same sort of smooth splitting type of break comparable to the shattered trunk of a lightening-struck tree.
A fracture that has a step-like surface, and shows a marked color difference from the remainder of the bone, is a postmortem break, one that occurred long after death. Postmortem breakage is commonly associated with small crumbs of bone that detach due to the dissolution and loss of collagen, the protein binder in bone. While perimortem bone breakage can occur in living and recently deceased individuals, it also can occur in very old bone that has been well preserved in dry desert caves and sands, in Arctic and Alpine permafrost, and in other such settings of very low biomechanical energy. Under these conditions bacterial and other agencies of diagenesis may be slowed down or even stopped altogether. Frozen mammoths and accidental mummification are well-known examples of the remarkable preservation that can occur, and which would allow perimortem-like breakage long after death.
Determining who the bone breaker was is more difficult than telling when the breakage occurred. Gary Haynes (2000) has cleverly argued that bones and tusks broken and polished, which appear to be products of human activity, were in fact done by elephant trampling at African water holes. Manifestly, context is critical but even so may be misleading. Bone breakage in an archaeological setting, where there is physical evidence of hyenas having also occupied the site, does not mean that all or even most of the breakage was done by humans. Observations of hyena chewing clearly reveal that they are capable of cracking open very large and heavy bones, just as well as can humans using bone or stone hammers. One such observation by Owens and Owens (1984:73) is representative. The event they observed was when a brown hyena, after cautiously ensuring herself that the killers, lions, had left the scene, approached and ate at the leftover carcass of a gemsbok:
After nibbling at morsels of stringy meat, tendons, and sinew, she opened her jaws wide and began to crush leg bones as thick as baseball bats and to swallow splinters at least three inches long. (We measured these later, by fecal analysis.) A brown hyena’s teeth are veritable hammers specialized for processing bone. The premolars are flattened and enlarged, unlike the sharp, scissorlike cutting blades of other predators. Tilting her head to one side, she wedged her teeth between the ball and socket of a hind leg until it tore free.
11 Postmortem breakage (see examples in various sites; see also Binford 1981). Bone breakage that occurs months or years after death often exhibits a step-and-ledge fracture surface that may be granular instead of smooth, and sometimes even crumbly or flaky due to the lack of collagen. Postmortem breakage occurs often in bones left on the ground surface, which even when mechanically undisturbed can exfoliate small flakes or large chucks. Old trampled bone usually shows postmortem breakage, and archaeological or paleontological excavation procedure sometimes causes postmortem breakage. Postmortem breakage should be expected in open sites because of the greater opportunity for weathering and collagen disintegration. Bone left on the ground or slightly buried is subject to exfoliation due to mineral crystals forming as a result of drying out after being wet in water containing dissolved minerals.
12 End-hollowing (see examples in various sites). This variable is a hallmark of carnivore chewing activity at the ends of long bones, ribs, vertebral spines, pelvic edges, and other parts of a skeleton where the cortex is thin. This chewing may remove most of an anatomical end or border, leaving the remainder with a cupped appearance, sometimes ragged, but often smooth and polished. Tooth marks are commonly nearby. End-hollowing is the result of carnivores attempting to remove marrow-rich spongy bone. We know of only one other agency that produces end-hollowing - the tumbling and abrasive action that bone can receive from wave action along a lakeshore, river, or in an energetic oceanic inter-tidal zone. Under these abrasive conditions, spongy bone is less resistant to erosive damage than compact bone, leading to differential loss and the formation of end-hollowing.
13 Notching (see examples in various sites; see also Binford 1981). We first observed this form of perimortem damage on the edges of fracture surfaces of bones from Razboinich’ya Cave. Because this cave’s larger occupants were mainly carnivores, we inferred that these notches were due to jaw and tooth pressure-flaking of one or more small pieces of bone at the tips of the carnassial molars and premolars responsible also for the bone cracking or fracture. These notches are usually large (>3 mm diameter), and common in big animal bones, making hyenas good candidates for their production. Since then, a few notches have been found suggesting human impact blows must have produced them with a bone billet or stone hammer. When a notch appears to have been caused by humans, that is, if the notched area shows the crushed appearance of hammer stone breakage, and if there are no nearby tooth dints, scratches, polishing, or end-hollowing, we assign the notch to human causality. Marean and Bertino (1994) suggested that spatial analysis of bone assemblages would be aided by focusing on midshaft fragments with hammer stone damage. In this way the effect of carnivore disturbance of the bone deposit could be subtracted and the hominid discard placement better defined. We do not disagree, but emphasize that distinguishing human and carnivore damage by a single criterion is not always possible. Cruz-Uribe (1991) would seem to agree.
14 Tooth scratches (see examples in various sites; see also Binford 1981). Slippage of a bone in the tooth-studded clamped jaws of a carnivore can produce scratches comparable to slippage scars left by a bench vice, the jaws of a pipe wrench, common pliers, and other gripping devices. These scratches usually have one deeper and straighter end, with the other being shallower and ending in faint drifting-curved or curved-and-recurved track. Nearly all have a U-shaped cross-section. We attribute fine and delicate scratches to small carnivores, whereas larger carnivores are thought to be responsible for broader and deeper scratches. We have observed such differences on bones chewed by modern small and large dogs.
15 Tooth dinting (see examples in various sites). Indentation pits caused by the surface penetration of pointed cusps of carnassial and other teeth are commonly seen with tooth scratches. These tooth dints are sometimes found in clusters of three or four, which vaguely record the shape of the responsible tooth. Occasional bone pieces, notably whole segments of a carnivore-ravaged long bone, will show dinting on both sides where the upper and lower jaws had gripped the bone. Like tooth scratches, we regard (1) small, shallow and (2) large, deep dints as having been left by small and large carnivores, respectively. We have recorded a few dints that could well have been produced by some unknown form of human bone processing. These rare examples are suspected of human causality because they occur near a hammered impact injury, and the pieces have no other signs of carnivore damage.
16 Pseudo-cuts (see examples in various sites). As the name we have given this variable suggests, these are cut-like perimortem features. They were originally observed in the Razboinich’ya hyena cave, the context of which indicates that they were very unlikely human-caused cut marks. Instead, they are mimics of stone tool cut marks left by carnivores, presumably the cave hyenas that utilized Razboinich’ya for thousands of years. Nearly all the pieces we scored as having pseudo-cuts also had one or more indications of carnivore processing. However, as Bunn (1991:445) notes, hyenas in particular are attracted to bone refuse left by African hunter-gatherers. They visit these kill or camp sites within hours of abandonment. Hence, both human and carnivore damage can occur on a piece of bone, which means that pseudo-cuts on bones in carnivore dens might have been on bone cut by humans elsewhere and later transported to the den.
Pseudo-cuts are straight, narrow and deep V-shaped gouges just like stone tool cut marks. They are not U-shaped and they do not wander about randomly like tooth scratches. They are not only like stone tool cut marks; they are also reminiscent of the very fine grooves that can be produced on bone with a freshly struck stone flake or burin. Conceivably, pseudo-cuts are the result of gouging by very sharp unworn teeth of a young animal, or by a cusp that had been chipped, leaving in a sense a kind of burinized edge. As of this writing, we have found no qualitative or quantitative microscopic difference between stone tool cut marks and pseudo-cut marks. Internally, both may even contain the hinge fracture remnants where minute bone shavings broke off within a cut mark.
17 Abrasions (see examples in various sites). Abrasions are an any-sized set of very fine closely spaced parallel striations that resulted from a bone slipping on an abrasive surface, such as having been placed on an anvil stone, or receiving a glancing blow from an implement with an abrasive surface, again stone, and again abrasive. Most sets of abrasions are associated with archaeological finds, although a few are not. The latter must then represent accidental trampling, slipping, or grinding on some manner of a finely abrasive surface, as limestone cave floors and mixed sand and icy beaches can be. While usually straight, a set of abrasion grooves may make a sharp turn due to additional slippage in another direction. Abrasions are scored by the number of parallel grooves that can be counted with a x20 hand lens on a given piece, regardless of the number of sets.
18 Polishing (see examples in various sites). Polishing occurs when a bone fragment is subjected to some manner of fine abrasive force that physically removes a very small amount of surficial bone, presumably the result of periostial gnawing. In so doing, the minutely rough surface is smoothed to a mirror-like condition that reflects light in a less scattered fashion than in the original state. We have observed this sort of polishing resulting from family dogs chewing on leftover BBQ steak bones. Polishing can occur any place where abrasive contact is possible. This most often is on the ends of fragments. We prefer to score polishing only on perimortem fracture surfaces. If polishing has occurred, the usually sharp fresh border is altered to a more rounded and reflective edge. We score end-polishing when it is present on one or both ends. Polishing on the middle of a piece can only be scored if there is a perimortem fracture present on one or both sides of a more-or-less rectangular fragment. When polishing occurs on both the end and middle, we refer to the condition as “both.”
The polished area can be as little as 0.5 mm in diameter (usually at a pointed end), and up to 10 cm or more in length (almost always along the middle portion). Polishing can be seen with a x10 hand lens, but we prefer x20. An assemblage may contain many pieces that are also extensively polished, as in the river-deposited Krasny Yar collection. This polishing is readily attributed to tumbling and scouring in an abrasive context. Very extensive polishing in a few pieces and less in others, as occurs in the Razboinich’ya hyena cave assemblage, suggests more-or-less random carnivore chewing. Low amounts of polishing are expected in low-energy settings such as swampy soil, permafrost, and rarity of humans. In archaeological sites with minimal carnivore presence but much polishing, some thought has to be given to trampling and the possibility of bag-boiling with the use of heated stones. The stirring of the bone fragment and adhering soft tissue porridge could create the polishing on any surface, whereas stirring the same mixture in a pottery cooking vessel can produce polishing only on the ends of fragments due to the geometry of the pot (White 1992). Finally, we think of polishing as an indicator of taphonomic activity, both perimortem and postmortem.
19 Embedded fragments (see examples in various sites). Small adhering pieces of bone situated in or at a notch, dint, tooth puncture, or impact point are referred to as embedded fragments. The frequency of embedded fragments in an assemblage is the combined result of the production agent (carnivores, humans, or both), and the predominant species and skeletal elements. We would in general expect more embedding by carnivore bone puncturing, especially in the ribs, pelvis, and ends of long bones of small - to medium-sized animals, than we would for human processing. Carnivore chewing is a relatively low-energy “squeezing” action, whereas human hammering is a relatively high-energy “smashing” action. Breaking an egg open by squeezing produces many times more incomplete hinge fractures than smashing it. Smashing produces many more completely separated pieces. For large animals we suspect the opposite effect, namely carnivore puncturing would be less due to bone density, so fewer embedded pieces should occur. Human smashing of big bones may lack the shattering power to produce unhinged fractures, so embedding would be more frequent. These expectations are based on an idealized homogeneous material, which of course is not the case for content or form of a carcass or skeleton. Nevertheless, we
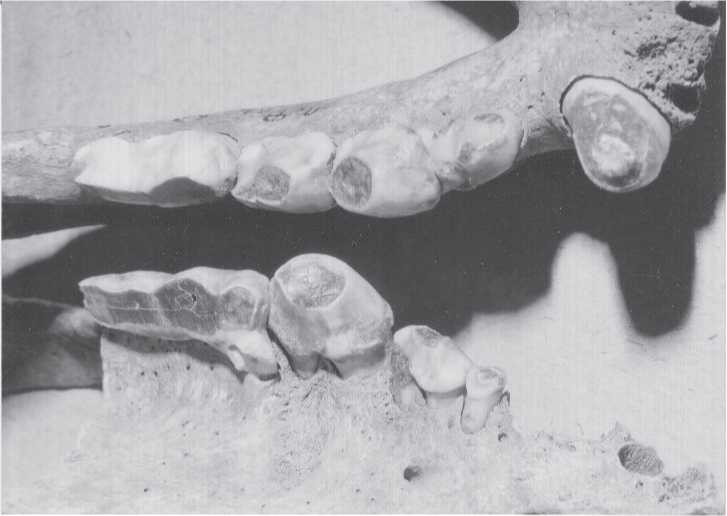
Fig. 2.12 Tooth wear. These hyena teeth of an animal estimated to be 15 years old illustrate the wear grade at which dentine is exposed but some degree of cusp anatomy remains. This photograph was taken to show that enamel thickness alone is not necessarily a strong indicator of diet. Hyena teeth actually have a rather thin layer of enamel, despite their bone-crushing dietary habit. Fanatic’s Cave (CGT color Krasnoyarsk Regional Museum 8-7-98:5).
Have scored embedding as a variable that we feel helps to identify the perimortem bone damage signature of large carnivores.
20 Tooth wear (Fig. 2.12). We added tooth wear to our variable list in the hope that it would provide useful information about prey age preference and seasonality. There is a great deal of natural history research on carnivore hunting behavior, especially wolves, but also hyenas and others. It was our hope that we could compare the ages of animals found in archaeological and paleontological sites to see if humans and carnivores hunted and scavenged in a similar fashion. Unfortunately, our selection procedures caused our samples of usable teeth to be too small for such an ambitious but secondary objective. In any event, we scored tooth wear using the same standard as used in the Arizona State University Dental Anthropology System (Turner et al. 1991): no wear = 0; dentine exposed = 1; cusps largely worn off = 2; pulp or secondary dentine exposed = 3; root stumps are all that remain = 4. Intermediate grades are such as slight wear but dentine not yet exposed = 0-1, etc. We considered an animal to be “young” when its tooth wear scored 0 or 0-1. Obviously, to achieve “real” ages we would have had to standardize by using only teeth of the same kind that erupted at the same time for most species, a precision far beyond the capability of our assemblages, even if we had assessed wear in every single tooth. Hence, this variable has proven to be disappointing except in the most general way, as shown in Table A1.20.
21 Acid erosion (see examples in various sites). Pieces of bone, teeth, and even small whole bones that have been swallowed by carnivores will be exposed to the destructive processes of part or the entire digestive track if not regurgitated before passing as feces. These acid-eroded pieces are commonly referred to in Russia as “stomach bones.” This term is more useful than, say, “gut bones.” The former allows for regurgitation, whereas once a bone or tooth reaches the gut it is not likely to be regurgitated. Destruction involves the dissolution, rounding, and polishing of bone or piece surfaces, be they spongy, compact, or both. As we have noted elsewhere (Turner et al. 2001b:26), 92 stomach bones from Dvuglaska Cave averaged about 4.0 cm in diameter, with a range of 1.7 cm to 7.5 cm. Presumably some percentage of very small stomach bones are eroded completely and leave no physical trace of their having ever existed, although the distinctive white color of hyena coprolites represents bone calcium residue. Because bone contains nutritionally useful collagen, powerful bone-crushing hyenas not only can gain access to energy-rich marrow, but also by accident and evolutionary adaptation extract protein from bone itself. Acid-eroded pieces are remarkably distinctive, and as such are one of our best indicators of carnivore presence, especially cave hyena presence. The occurrence of stomach bones in an archaeological site signals discontinuous human occupation, as well as probable stratigraphic disturbance due to the digging behavior of hyenas and other carnivores. Observers of hyena behavior regularly note that these creatures regurgitate parts of their meals, both as a means to rid themselves of huge undigestible “hair balls,” and as a way to feed pups. A description by van Lawick and van Lawick-Goodall (1970:248) is insightful:
Hyenas are frequently sick [vomit], and always they roll in it. It was not for some while that I realized that, for the most part, the hyena is not being sick in the normal sense of the word, but is actually regurgitating a mass of undigestible hair. Often, before or after rolling on this hair mass, a hyena picks out fragments of partially dissolved bone - when the hyena chews on them it seems that they are soft for there is no sound.
22 Rodent gnawing (see examples in various sites). Finely chiseled, flat-bottomed, parallel tooth grooves, particularly at a sharp, bony edge, are unmistakable evidence of rodent activity. Some of their bone gnawing is nutritionally motivated to extract grease, minerals, and trace elements, but some gnawing probably serves other purposes such as controlling the size of the continuously erupting incisors. Despite the ease of recognizing and scoring this variable, it has not proven to be an especially valuable perimortem taxonomic trait because of its generally infrequent occurrence in our Siberian assemblages. However, we do consider rodent gnawing to be an indicator of site vacancy or abandonment (Table A1.22).
An interesting experiment and excellent literature review by Meg Thornton and Jennifer Fee (2001) found that in 150 skeletal elements belonging to four species (deer, squirrel, raccoon, and chicken), parallel grooving left by domesticated mice occurred in only 17 pieces. This amount was only about 10% of the total damage done by the mice. The other and more frequent damage included missing pieces and roughened areas. In our study, roughening and missing pieces could just as well have been done by carnivores. Hence, while our criterion for rodent damage is reliable, the actual frequency was likely greater.
23 Insect damage. Small holes and surface traces are sometimes left in bone by burrowing insects. These traces can be distinguished fTom root damage by the latter’s branching dendritic pattern with older and wider branches thinning down to younger and narrower branches. As was noted in 2001 (Turner et al. 2001a:27), the identity of these insects, and possibly some other form of invertebrate, remains unknown to us.
24 Human bone and teeth. This special variable considers whether humans have damaged human bone. The damage forms include cutting, perimortem breakage, abrasion, polishing, burning, and (many) missing vertebrae. If these six traits are identified in an assemblage of human bones, then cannibalism can be suggested.
25 Cut marks (see examples in various sites). Inasmuch as we consider stomach bones to be a major taphonomic characteristic of hyenas, cut marks are similarly important as indicators of human carcass processing. Stone tool cut marks are generally V-shaped in cross-section due to their being the result of a slicing action. Within a cut itself there can be ledges, hinge fractures, and other irregularities. These can often be seen with a x20 hand lens, although a x75 dissecting microscope reveals these minutia much better. Cut marks result when stone knives, blades, or flakes used for butchering, dismemberment, and tissue slicing accidentally cut into underlying bone. As indicators of butchering, cut marks are often found near anatomical joints, indicating dismemberment of limbs, heads, jaws, and other body parts. Cut marks are variable in length, breadth, and number in a set. A set presumably represents a repeated effort to dismember a body part, to remove a muscle mass, or to separate hide fTom underlying muscle. We have found as many as 200 cut marks on a single piece, although most cut pieces have only two or three cut marks. Paul Volkov (2006) has written and illustrated a marvelous analysis of stone tool production technology; it seems from our reading that there are few differences in the resulting cut marks of stone tools. The goal of the various ways of making stone cutting tools is to produce a slicing function. In the early months of our study we tried to identify a given cut mark with a particular form of stone tool. We were unable to recognize any macroscopic variation that we could link to time period (tool type) or location (stone types). Perhaps we should have examined cut marks more closely, but our goal was more broadly focused. We did not distinguish deep cut marks from shallow cut marks. However, we attributed this difference to the act and actor of butchering, not to the form of the cutting tool.
26 Chop marks (see examples in various sites). Whereas cut marks are produced by a slicing or back-and-forth sawing action, chop marks are produced by hammer or hatchet impact action. The result is a round and sometimes deep crater. If the hand axe, heavy flake, or worked core is dull, the chop mark may have rough and ragged internal surfaces. If the chopping edge is sharp, the internal surface can be smooth like a cut mark, but wider and non-linear, and can often show smashing at the bottom of the crater. Some small percentage of chop marks are likely due to a bone having been pounded on a rough rocky surface or anvil stone. The resulting craters are indistinguishable from those produced by a stone tool. We have seen a couple of chop marks that seem more likely to have been produced by hyena teeth than by stone tools.